The inner debris disk at the heart of the Epsilon Eridani planetary system has long puzzled astronomers. A team of Steward Observatory researchers is redefining how we study it.
Steward Observatory’s Sai Krishanth is lead author on a new paper using Hubble data to define the deepest limits on scattered light emission from the Epsilon Eridani inner debris disk.
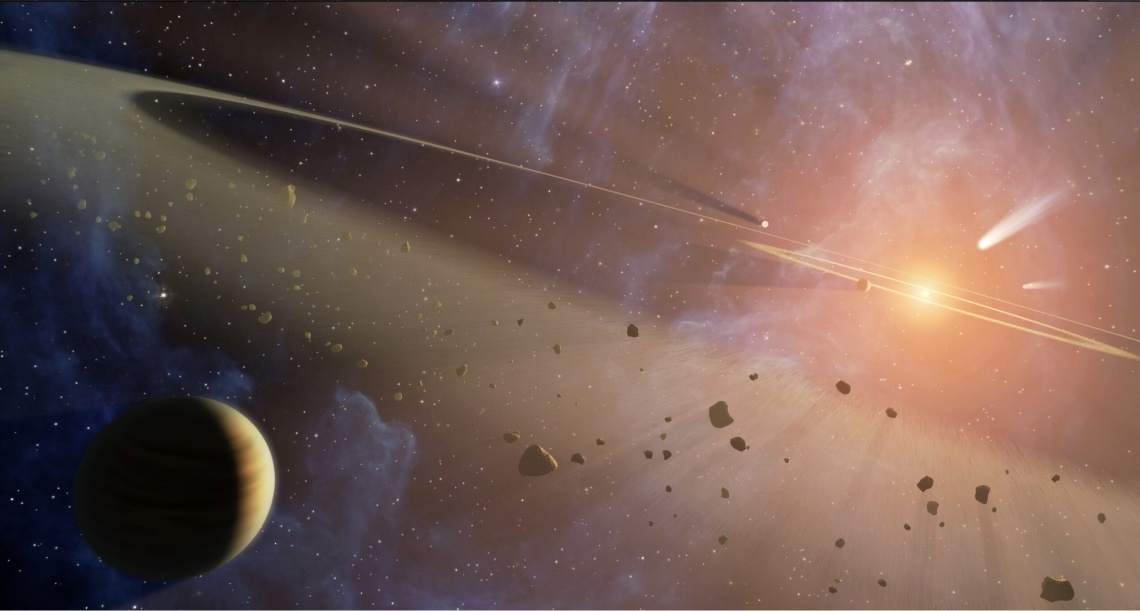
Image: artist's impression, showing asteroid belts and a planet orbiting Epsilon Eridani, NASA/JPL-Caltech
This month, a paper in the Astronomical Journal authored by Sai Krishanth—along with Steward Observatory astronomers Ewan Douglas, Ramya Anche and Justin Hom—set out to directly image the elusive Epsilon Eridani inner debris disk. The team came away with a clearer understanding of the composition and brightness of the disk—and an innovative method for studying it.
The star Epsilon Eridani, one of our closest galactic neighbors, has intrigued astronomers for decades. The star is less than a billion years old: around the same age our own solar system was when life first began to form on Earth. Science fiction writers have long imagined fictional worlds circling Epsilon Eridani, from the 1990s Babylon 5 TV space opera to Alistair Reynolds’ Revelation Space novels woven with elements of Lovecraftian horror. Like our own Sun, Epsilon Eridani is orbited by an enormous Jupiter-like gas giant, and by an outer asteroid belt of comets—similar to our solar system’s Kuiper Belt. Infrared evidence also shows that the solar system has two other inner belts, filled with debris that could gradually accrete to form larger bodies. It’s a prime laboratory for understanding how planets form around Sun-like stars—with one hiccup: the inner debris disks around Epsilon Eridani have never been seen.
Although Epsilon Eridani is only 10.5 light years away—close enough to see with the naked eye—the inner secrets of the solar system have remained elusive. In the 1980s, NASA sent IRAS—the first infrared telescope ever sent into space, with instrumentation aboard it designed by Steward Observatory’s George Rieke—to survey the sky. IRAS was the first telescope to discover “debris disks”—diffuse bands of rock and dust that are likely remnants from planet formation. In some regards, IRAS discoveries were the birth of exoplanet science. The telescope was discovering evidence of planetary formation in other solar systems nearly a decade before the first exoplanets were discovered orbiting a pulsar in the constellation Virgo. IRAS identified four debris disks, still known as the original “Fabulous Four,” including the outer disk around Epsilon Eridani.
Since then, astronomers have puzzled over the star system using ground-based and space telescopes, from the Large Millimetre Telescope to Spitzer. These earlier studies indirectly confirmed the presence of two other inner debris disk around the star; using the Hubble Space Telescope, Krishanth’s team hoped to finally find direct visual evidence of the innermost disk. While their study yielded no visuals, it offered the deepest-yet understanding of what the disk will look like when astronomers do find it at last.
Using a light-blocking instrument called a coronograph, Krishanth and his team managed to set constraints for what the disk could—and could not—look like. They studied the inner disk’s albedo: the measure of how well the dust grains reflect light. While previous papers suggested that the inner disk might be a ring, Krishanth determined that it is likely more diffuse, made of fine particles less than a micron in size—a hundred times smaller than a grain of sand, and tinier than the human eye can see. Albedo also gives astronomers clues about the composition of a disk. Because the inner disk of Epsilon Eridani had low albedo, the team could discount the possibility that the disk is composed of ice, since frozen water is very reflective. Instead, the composition of the disk is likely what the team calls “typical rocks”—less reflective particles like silicates and carbon grains. This is true in our own solar system, too—much of the dust that exists between planets is carbon-based.
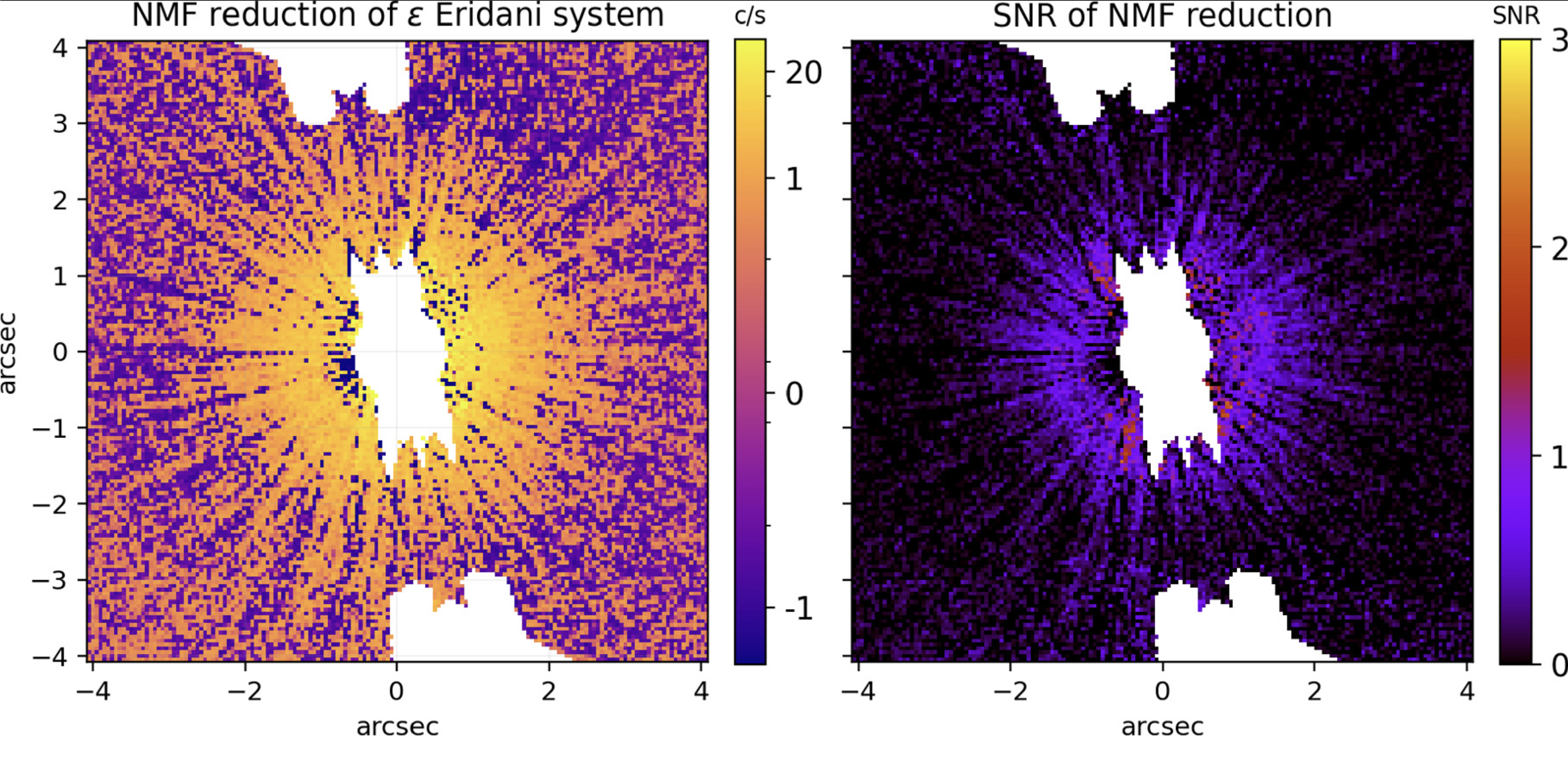
The NMF processed result.
The team also established a ceiling for how bright the disk could be: they processed their coronograph data finely enough to nullify the starlight by a factor of 100 million; this means, says Krishanth, “the disk cannot be brighter than 100 million times fainter than the star.” Ultimately, these various “ceilings” that the team found show that their questions simply cannot be answered even by today’s top-tier technologies. But, says Krishanth, “with the power of the upcoming Nancy Grace Roman telescope, we should be able to reach a sensitivity of a billion times fainter than the star.” Krishanth and many other astronomers from Steward Observatory are working on testing and developing the equipment that this next-generation space telescope will carry. When the Roman Space Telescope launches, the team will be poised to finally answer some of the questions they sought in this current paper.
However, the paper does more than lay the stage for new imaging technology on the horizon. It also opens up a sophisticated new approach to processing coronograph data—an approach that is specifically targeted at studying debris disks. Coronagraphs like the one on Hubble are essentially high-tech attenuators that are intended to block out starlight so that fainter objects around a star can be detected. Some light still manages to escape past the coronograph, and astronomers use post-processing algorithms to clean up the image and get rid of extra starlight. However, Krishanth says, the algorithms currently used are optimized to find planets, not dust. “They’re really aggressive in how they go about cleaning the image.” Imagine editing a photo on your phone, and sliding the clarity filter all the way up. Big objects remain sharp, but smaller details in the picture get wiped out.
To remedy this, Krishanth’s team used a newer technique called Non-negative Matrix Factorization, which had been used initially in a 2018 paper on debris disks. The technique works spectacularly for dim, diffuse objects like debris disks, but astronomers have been slow to adopt it because the time it takes to run the algorithm is prohibitive. Krishanth’s team modified their approach, rewriting the disk method to run on a graphics processing unit instead of a time-intensive central processing unit. “Instead of going through a very complicated pipeline,” Krishanth says, “you go through a very simple, much faster pipeline that can massively accelerate a computation.”
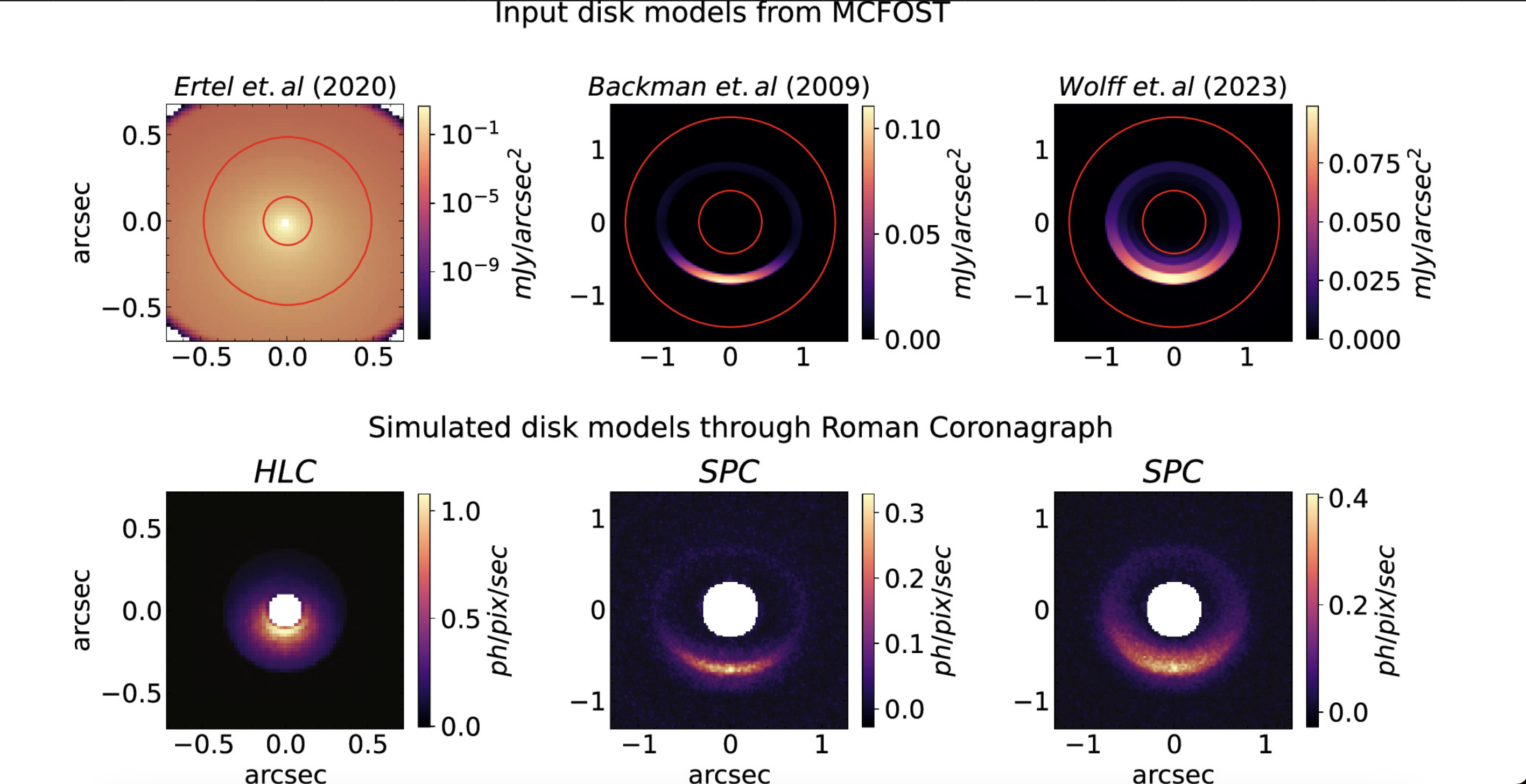
Simulations of the disk through the Roman telescope.
The paper marks a new chapter for investigating debris disks with an algorithm designed specifically for the task. When astronomers finally turn the new coronagraph aboard the Roman Space Telescope toward Epsilon Eridani, the long-awaited first images of the inner debris disks may well be processed by the technique refined by Krishanth and his team.